In general, the behavior in the wake of a wall-mounted circular cylinder with finite height is considerably different from that of two-dimensional bluff bodies. Unlike the flow field associated with a conventional 2D cylinder, a cantilevered finite-span cylinder is largely influenced by the presence of a spanwise (i.e., along the height of the cylinder) velocity component, most notably the downwash issued from the free end of the cylinder. A very important distinction between 2D and 3D cylinders is the degree of interaction between the downwash and the near-wake structure, which is dependent upon the aspect ratio, AR (i.e., height-to-diameter ratio). However, the flowfield associated with finite span, wall-mounted cylinders require focused attention not only on aspect ratio, but also the cylinder’s height with respect to the local boundary layer thickness. The main focus of this proposal is to investigate the mechanisms, which drive these constituent vortical structures, formed in the flowfield of a wall-mounted, low aspect ratio cylinder submerged in a laminar and/or turbulent boundary layer. This leads to further exploration of one of the most notable difficulties in studying finite span cylinders: the three-dimensionality.
Some of these three-dimensional flow behaviors have been reviewed in the literature, which characterizes the mean flow in the near wake of a low-aspect ratio circular pin by three dominant features: (i) a horseshoe (necklace) vortex system that forms due to the interaction of the incoming boundary layer with the pin and wraps around the sides, developing into two counter-rotating streamwise structures in the wake, (ii) the arch-type vortex, which is due to the combination of separation of the flow from the sides of the pin and the downwash at the free end of the pin, and (iii) tip vortices that emerge from the pin’s free end.
The proposed work attempts to expand the existing envelope of knowledge and understanding of the flow physics of finite-span, low aspect ratio cylindrical pins. The target for this research is to investigate how the passive and/or active surface modification actuators introduce vorticity and momentum as a means of energizing the boundary layer to delay or mitigate separation altogether. In order to optimize the actuators for specific flow conditions, the dominant mechanisms which contribute to the modification of the flow field must be well understood. This work aims to conduct detailed studies of the flow physics associated with the interaction of a low aspect ratio cylinder in a cross-flow, where the height of the pin is similar to the local boundary layer thickness, and corresponding three-dimensionalities, which characterize the complex wake structure. Through this work, increased depth of knowledge will be attained to provide new approaches to flow control systems by incorporating passive, active, or hybrid (combination of passive and active components) control techniques optimized for the given system and performance requirements.
The parameters were selected such that the cylindrical pin(s) imposes minimal drag losses (i.e., submerged in the local boundary layer). In addition, the incoming flow conditions and pin geometry were selected based on the characteristic instabilities present. The dynamic pin was designed such that the piezoelectric driver, which provided physical excitation of the pin itself (wall-normal oscillations at prescribed amplitude and frequency), would be able to reach frequencies equivalent, or subharmonic, to the frequencies of the distinctive vortex shedding street. The objective of these chosen parameters is to compare the interactions of a static and dynamic pin in terms of the local pressure drag losses and production of turbulent kinetic energy. Under dynamic conditions, the pin would be able to increase fluid mixing in the wake, entraining the mean flow into the boundary layer and thus energizing it through forced lock-on of the shedding vortical structures. The dynamic pin is designed to take advantage of the natural instabilities of the flow field surrounding the finite-span pin by amplifying the size and strength of the vortical structures to enhance fluid mixing, reduce local pressure drag, and overcome separation.
From this study, the flow physics associated with finite-span, low aspect ratio cylinders will be further understood, providing more insight into the mechanisms of the interactions between the three-dimensional behaviors to attain a means of quantifying these devices and the advantages they offer as flow control actuators. This will ultimately lead to the identification of new techniques of manipulating the global flow field via localized modifications which may pose significant influences in new system designs for aerodynamic, underwater and land vehicles, or heat transfer applications.
Dynamic Pin in a Cross Flow
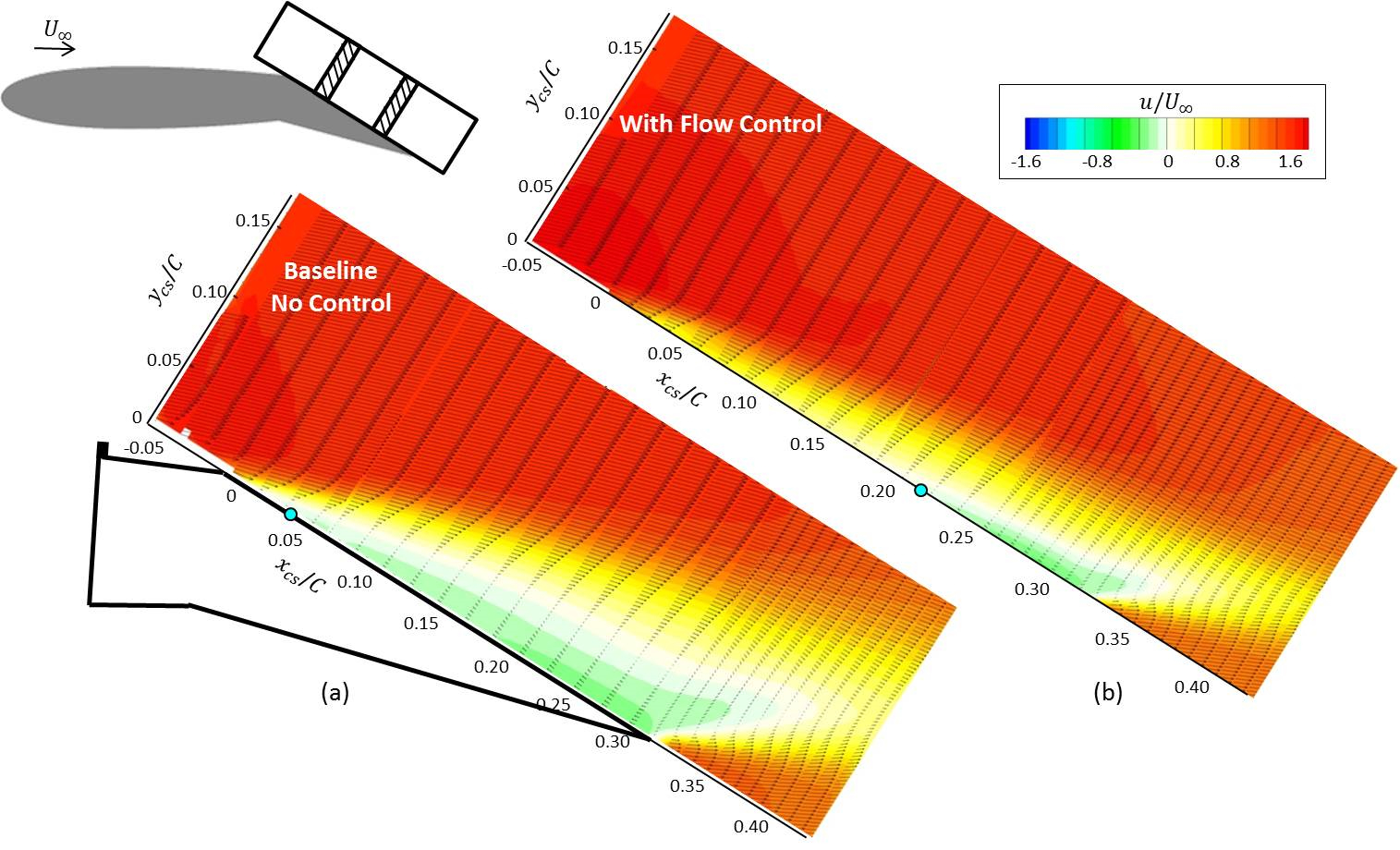
Figure 1: Normalized, mean streamwise velocity contours along the centerline of the deflected control surface of a modified NACA 0012 model for the (a) baseline case, and (b) an array of static low aspect ratio pins placed upstream of the hingeline.
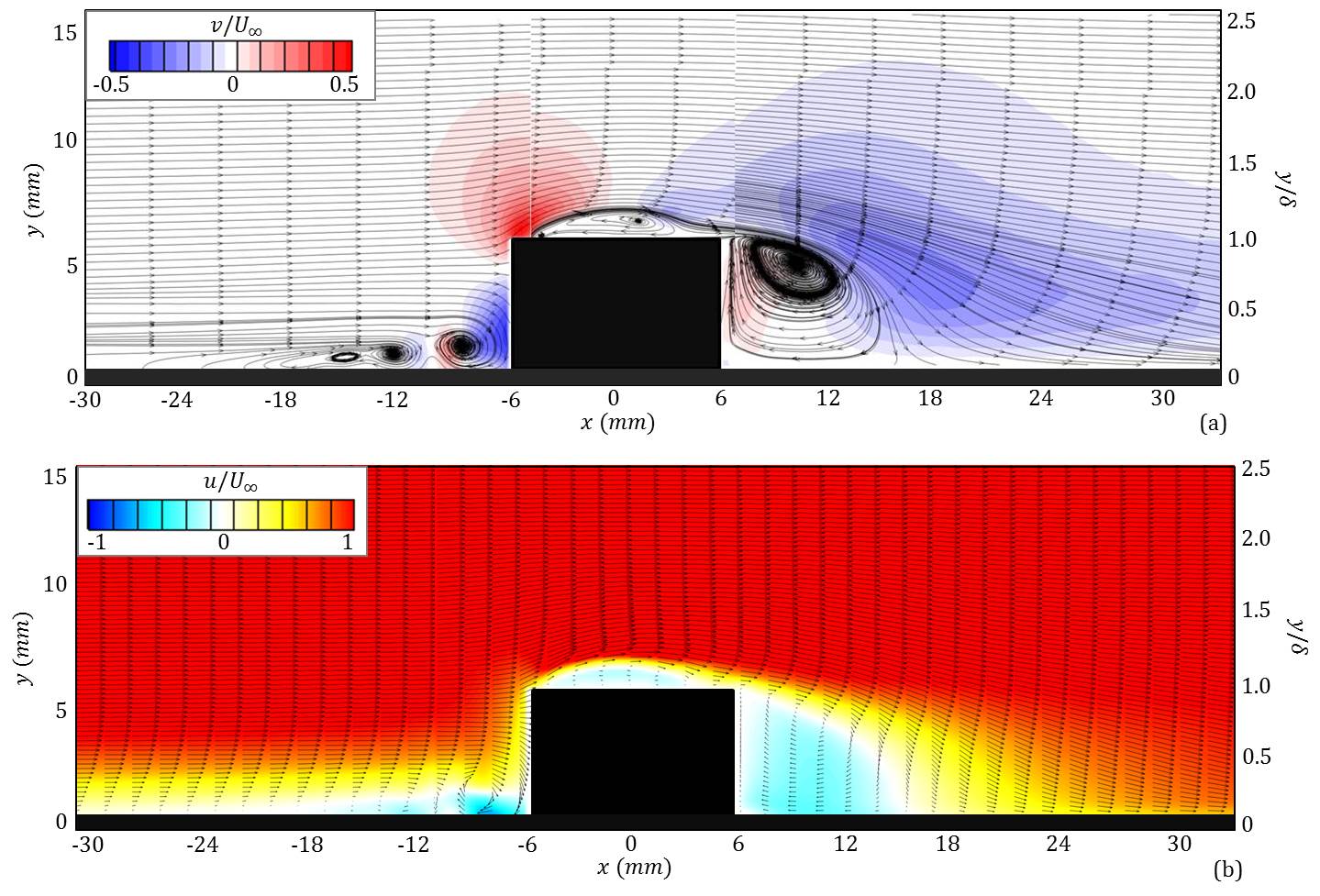
Figure 2: Contours of normalized, mean (a) wall-normal velocity superimposed with streamlines and (b) streamwise velocity superimposed with velocity vectors along the centerline of a static low aspect ratio cylinder in a laminar boundary layer.
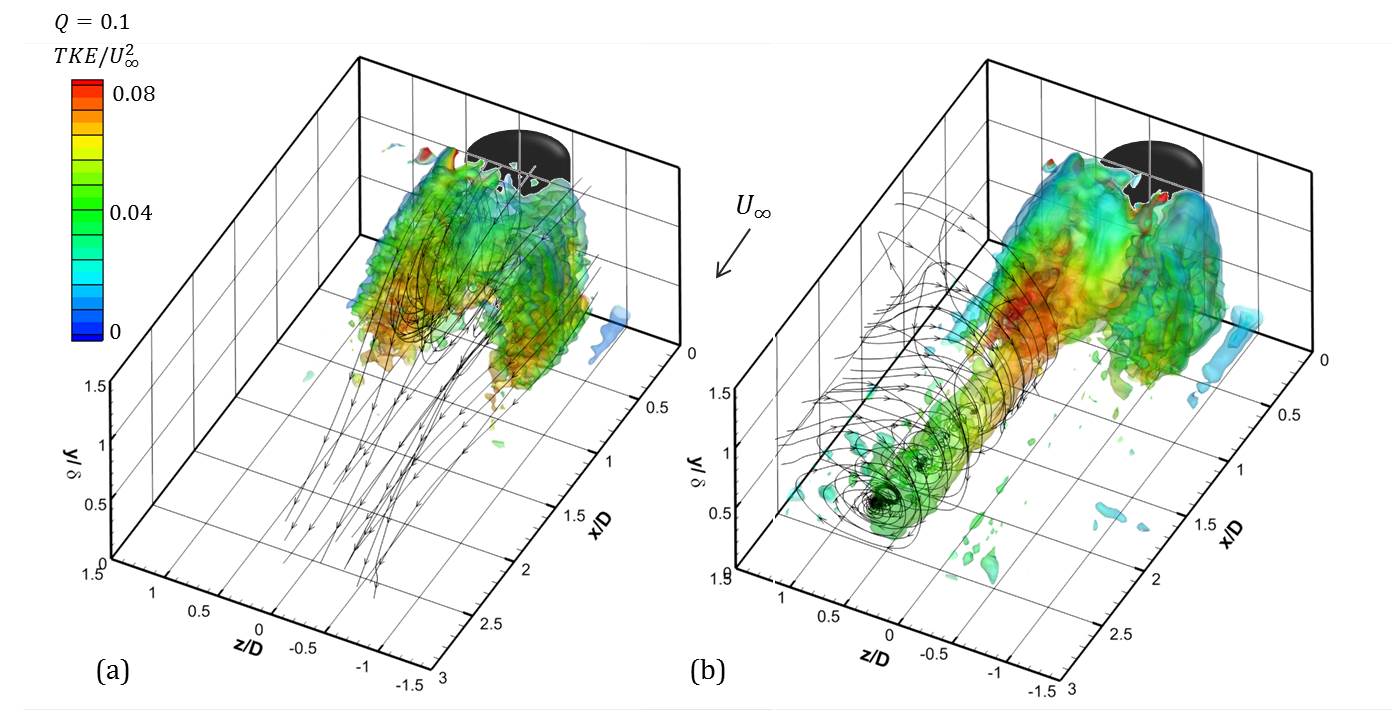
Figure 3: Iso-surfaces of time-averaged, normalized Q-criterion, colored by normalized TKE for (a) the baseline flow (no jet actuation) and (b) synthetic jet actuation at fj = 1800Hz.
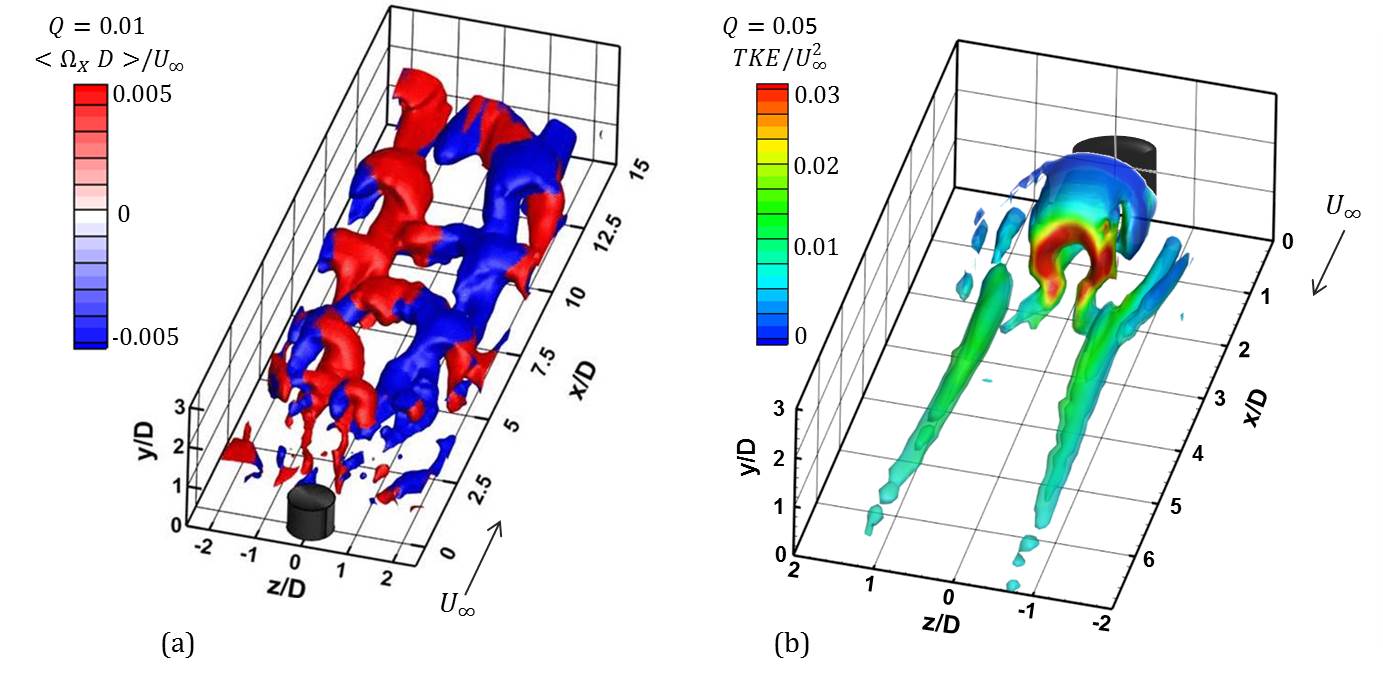
Figure 4: Iso-surfaces of (a) phase-averaged, normalized Q-criterion, colored by streamwise vorticity at a single phase along the dynamic cycle (ϕ=0°), and (b) time-averaged, normalized Q-criterion, colored by normalized TKE for the static pin.
Related Publications:
- Gildersleeve, S., Tuna, B.A., and Amitay, M., "Interactions of a Low Aspect Ratio Cantilevered Dynamic Pin with a Laminar Boundary Layer," AIAA Journal, July 2017.
- Gildersleeve, S., and Amitay, M., "Control of Flow Separation over a Flapped Airfoil using Low Aspect Ratio Circular Pins," submitted to AIAA Journal, May 2017.
- Gildersleeve, S., and Amitay, M., “Virtual and Physical Surface Modifications as Means for Flow Control”, submitted to AIAA Journal, July 2017.
Conference Papers:
- Gildersleeve, S., and Amitay, M., "Three-Dimensional Wake Characteristics Associated with the Jet Assisted Surface Mounted Actuator, " Aviation 2018, June 25-29, Atlanta, GA, 2018.
- Gildersleeve, S., and Amitay, M., "Dynamic Pin Actuator and its Application for Separation Controll," 57th Israel Annual Conference on Aerospace Sciences, Tel-Aviv & Haifa, Israel, March 15-16, 2017.
- Gildersleeve, S., Amitay, M., and Clingman, D., "Flow around Low Aspect Ration Cylinders and their Applications, " 55th AIAA Aerospace Sciences Meeting, AIAA SciTech Forum, Grapevine, Texas, AIAA 2017-1449, January 2017.
- Gildersleeve, S., Clingman, D., and Amitay, M., "Separation Control over a Flapped NACA 0012 Model using an Array of Low Aspect Ratio Cylindrical Pins, " Aviation 2016, June 13-16, Washington DC, 2016.
- Gildersleeve, S., Tuna, B. A., Leong, C. M., Clingman, D., & Amitay, M. (2015). Interactions of a Low Aspect Ratio Dynamic Pin with a Laminar Boundary Layer. In 45th AIAA Fluid Dynamics Conference (p. 2634).